An Overview of the Materials Used in Additive Manufacturing in Dentistry
Neil T. Griseto, BDentSc, MS
During the past decade, extraordinarily rapid developments in additive manufacturing have resulted in greater adoption of 3D printing in the dental industry and, simultaneously, a lack of validation regarding workflows and materials. Additive manufacturing is "the process of joining materials to make objects from 3D model data, usually layer upon layer, as opposed to subtractive manufacturing methodologies, such as traditional machining," as defined by ASTM International.1 Additive manufacturing holds promise for dentistry because it has the capability to create forms that can intimately fit intraoral structures, such as prepared teeth, without being limited by the drawbacks of tool sizes or toolpath restrictions that can be encountered when using subtractive manufacturing methods.
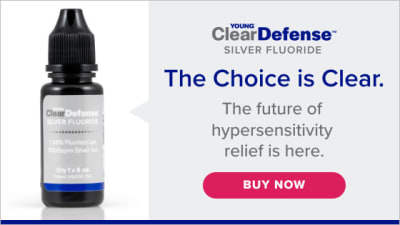
Young® ClearDefense™ Silver Fluoride
The additive manufacturing processes with applicable materials that are typically used in dentistry include vat photopolymerization and powder bed fusion. Vat photopolymerization is the method by which a light source is used to cure photopolymer resin in layers on a build plate that is incrementally raised from or lowered into a container of uncured liquid resin for the deposition of each subsequent layer. Several variations of this technology exist, including stereolithography (SLA), digital light processing (DLP), continuous liquid interface production (CLIP), and more. Powder bed fusion is a process in which thermal energy is used to selectively fuse particles of a powdered material together.2 Alternatively, in a process known as binder jetting, a liquid bonding agent can be selectively deposited onto a powdered material to join the particles of the material together, followed by removal of the binder and sintering or infusion.2
Additive manufacturing can involve a single-stage process in which the intended geometry and material properties of the object are achieved in one step or a multistep process. Multistep processes usually involve achieving the intended geometry of the desired object with additive manufacturing, but the intended material properties are realized in a second step, such as high temperature sintering in a furnace or investment casting.2 In dentistry, the additive manufacturing of hybrid ceramics and metals can involve multistep processes. For example, when a resin matrix with embedded ceramic particles is vat photopolymerized, the process is followed by sintering to remove the resin and fuse the ceramic particles to form a crown.3
The materials that are used in additive manufacturing in dentistry can generally be divided into four categories: polymers, metals, ceramics, and composites.
Polymers
Of the 3D printing materials used in dentistry, polymers are perhaps the most widely available, and they can be leveraged to fabricate a variety of models, patient-specific devices, and appliances.
Models
Some of the most beneficial uses of additive manufacturing in dentistry are for modeling and rapid prototyping. Commonly, this is achieved through vat photopolymerization using methacrylate, epoxy, and functionalized vinyl ether resins.4 A light activator is included that is sensitive to the specific wavelength of light used in the additive manufacturing process, which is particular to each different brand of printer.5
The desirable properties of materials used in the additive fabrication of models are similar to those of gypsum casts that reproduce the structures of the oral cavity, including trueness and precision, dimensional stability, temperature resistance, and abrasion resistance. Trueness and precision are dependent on the technology used to capture the object being reproduced and the additive manufacturing technology used to cure the resin (eg, SLA, DLP); however, dimensional stability, temperature resistance, and abrasion resistance are dependent on the material used. Over time, additively manufactured models may not remain dimensionally stable, even if they are stored in conditions where they are not exposed to light or heat.6
Surgical Guides
Due to the fact that surgical guides are exposed to the oral environment and surgical wound sites, they have an additional requirement of biocompatibility. The uncured methacrylate polymeric resin components of additively manufactured surgical guides can be considered skin, eye, and respiratory irritants.7 As a result, surgical guide resins are required to undergo a multistep manufacturing process involving post-processing procedures to ensure maximization of the material properties and a reduction of residual monomer. Post-processing procedures for a 3D printed surgical guide include exposure to solvents, heat, and light for a specific time and intensity followed by sterilization before it can be considered a medical device appropriate for its intended use.8
Occlusal Guards
The desirable properties of materials used to fabricate occlusal guards include adequate hardness, excellent wear resistance, low water sorption, high dimensional stability, ease of repair and modification, and low cost. Materials specifically designed for this purpose have become available for the additive manufacturing process. Unfortunately, guards made from these materials do not exhibit the same combination of desirable properties as conventionally injection molded, heat processed, or subtractively manufactured guards when studied in vitro.9 However, this is counterbalanced by the lower cost of resins for additive manufacturing when compared with subtractively manufactured materials and the ease of refabrication when compared with that of conventional processes.
Orthodontic Appliances
The primary workflow utilized to manufacture orthodontic appliances, particularly for sequential aligner therapy, is a multistep process involving 3D printing a model and then using it for the thermal/pressure forming of polymer sheets. The direct printing of aligners is challenging due to a lack of available 3D printing materials that mimic the properties of the existing non-additive manufacturing polymer sheets used for this purpose. However, some success has been demonstrated by using the 3D printing materials intended for occlusal guard fabrication, and it has been suggested that there may be some benefits to direct printing when compared with the thermal/pressure forming processes, including the ability to control the loads to specific teeth by precisely varying the thickness of the material.10 This type of customization is possible with thermal/pressure forming workflows, but it is more challenging and labor intensive because it requires manual modification of the aligners. In addition, direct additive manufacturing processes could help to reduce waste by eliminating the need to print models. Currently, there is no available waste management system for recycling photopolymerized additively manufactured models.
Dentures
The use of additive manufacturing for the fabrication of dentures typically requires two resins—one for the base and one for the teeth. Alternatively, it is possible to print a complete denture with a single resin that is tooth colored and then characterize the base with pink composite after post-processing is completed. The desirable properties of a material to fabricate dentures are similar to those for occlusal guards because they are subjected to the same requirements and environment. Digital dentures should also be esthetic, cost-effective to manufacture, and easy to repair or replace.
A review of the topic of additively manufactured dentures showed that conventionally processed and milled denture bases were superior regarding fracture toughness, flexural strength and surface hardness, whereas additively manufactured denture teeth were similar to conventional ones regarding wear resistance and fracture resistance. Furthermore, the bond strength of conventional denture teeth bonded to heat-processed denture bases was higher when compared with that of conventional denture teeth bonded to milled or 3D printed bases.11 The same review also found that patients' esthetic outcomes were worse for CAD/CAM dentures (ie, additively manufactured or milled) when compared with conventionally manufactured dentures but that the workflow generally required fewer appointments.
Metals
The fabrication of metal prosthetic components or restorations using additive manufacturing can be accomplished using a single-step process (ie, direct printing) or a multistep one (eg, printing followed by burn-off and sintering or printing followed by lost wax investment casting).
The single-step process involves powder bed fusion in which an energy source that is either a laser or an electron beam is used to melt particles of metal and fuse them together. Like with other additive manufacturing technologies, objects are built layer by layer. In the single-step process, the object is printed in its final dimensions but has supports that need to be removed or surface roughness that requires finishing. This process is most useful in the creation of metal copings for full-coverage ceramometal crowns, frameworks for removable dental prostheses, and bars or other metal substructures for full-arch implant restorations. The technology produces metal parts with greater density and fewer defects than those produced using traditional casting methods, and it has a reduced fabrication time and the potential to minimize human error.12
The multistep process for additively manufacturing metal restorations may include binder jetting technology or the CADcast technique. In the binder jetting process, the printed object is either sintered or hot isostatically pressed in order to densify the metal, remove the polymer, and create the final object. Unlike selective laser melting, this process does not require the creation/removal of supports.13 The CADcast technique relies on vat photopolymerization for the first stage, and then its second stage includes investment, burn out, and conventional casting. In one study, when electron beam melting, selective laser melting, and CADcast techniques were compared in the fabrication of metal partial denture frameworks, CADcast was found to produce the truest results with the least surface roughness prior to surface finishing.14
Ceramics
Zirconia, lithium disilicate, and feldspathic porcelain restorations can all be produced using additive manufacturing. The processes available on the market are typically multistep and primarily use vat photopolymerization and binder jetting as methods. In the vat photopolymerization process, a slurry of resin and dry ceramic material is mixed and used to print the restoration. The resin behaves as a binder, which is removed prior to sintering to create the final object. Binder jetting, as previously discussed, requires a secondary debinding and sintering process to achieve the desired material properties. These methods have not been commonly adopted in dentistry, and there is scant evidence in the literature about the advantages or disadvantages of additive manufacturing in the fabrication of all-ceramic restorations. Research indicates that subtractive manufacturing methods produce restorations with superior accuracy and material properties.15
Composites
Additive manufacturing holds significant promise for the fabrication of indirect composite restorations. Since the introduction of vat photopolymerization technology to dentistry, the commonality between it and direct composite resin technology has been of interest. What is particularly desirable about the use of additive manufacturing techniques to fabricate definitive restorations is that unlike subtractive techniques, they can be used to create shapes that can be intimately adapted to the irregular geometry of extracoronal and intracoronal restoration preparations. They are also more cost-effective and more time efficient. To that end, the materials in use for temporary and definitive restorations in dental additive manufacturing are largely composites.
The ninth edition of The Glossary of Prosthodontic Terms defines composite resin as "a highly cross-linked polymeric material reinforced by a dispersion of amorphous silica, glass, crystalline or organic filler particles and/or short fibers bonded to the matrix by a coupling agent."16 Based on the descriptions of some of the materials presented earlier in this article, they could be included in this definition in their pre-sintered states, which can lead to some confusion. Composite resins exist on a spectrum based on their inorganic filler content, and since a 2023 update to the American Dental Association's Current Dental Terminology, resins that contain greater than 50% filler content are considered to be ceramics, even if they are 3D printing resins.17 Regardless of the terminology used, the primary concern of dentists should be for the behavior and long-term performance of these materials, and unfortunately, there is scant data available—short-, medium-, or long-term—because of how recently these materials have become available. The data that is available is in vitro, and it shows that when similar polymeric materials are used, additive and subtractive manufacturing methods produce similar results regarding mechanical properties with the exception of flexural strength and flexural strength after aging in a chewing simulator.18
Conclusion
Relative to other methods, additive manufacturing in dentistry is in its infancy; however, the technology can be applied to a very broad range of materials and shows great promise. When the limitations of the currently available materials are overcome, 3D printing will likely become the method of choice for the fabrication of dental restorations due to the relatively low cost of printers and materials, more efficient workflows, and reduced treatment times.
About the Author
Neil T. Griseto, BDentSc, MS
Diplomate
American Board of Prosthodontics
Fellow
American College of Prosthodontists
Private Practice
Oakbrook
Terrace, Illinois